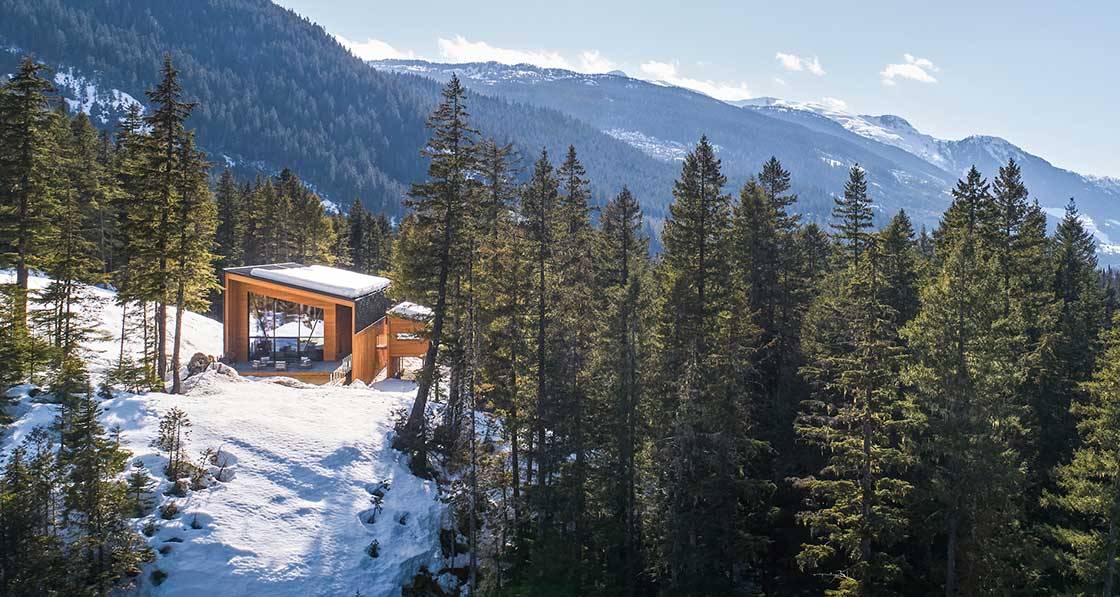
- Big picture
- Posted
Big picture - off grid passive house in British Columbia
Issue 43 featured an off-grid prototype house in British Columbia, designed and constructed to demonstrate an innovative approach to future building.
1. Introducing SoLo
This article was originally published in issue 43 of Passive House Plus magazine. Want immediate access to all back issues and exclusive extra content? Click here to subscribe for as little as €15, or click here to receive the next issue free of charge
Located in SoLo Valley in the Coast Mountains north of Whistler, British Columbia, SoLo is an off-grid prototype designed and constructed to demonstrate an innovative approach to future building – by generating its own energy and minimizing its carbon footprint.
SoLo fulfills a brief both as a single-family home and as a corporate retreat and prototype intended to demonstrate an approach in how to build future alpine settlements in British Columbia that will adapt to extreme weather patterns.
Now more than ever, in the face of the current climate crisis and depleting natural resources, we must choose to build differently and expect buildings to counteract their negative consequences. SoLo investigates a path forward through its deliberate choices and priorities. The project started with an overarching set of principles and goals that would lead every decision: eliminating harmful materials used in both construction and finishes; minimizing energy consumption through a high-performance building enclosure system, appliances, and fixtures while also generating energy on site; and reducing greenhouse gas emissions, both in operation and embodied in the construction.
2. An ‘enclosure first’ approach
The design allows the inhabitants to celebrate the natural beauty of the valley and provides an immersive connection with the raw beauty of the surroundings.
A platform on stilts raises the house up off the ground, minimizing the footprint and amount of excavation required on the landscape, as well as reducing the need for concrete. Mass timber is used to create a compact, pure form for habitation, with the CLT serving as both a structural element and interior finish for walls, roof and floors, reducing the need for interior materials. Given the valley’s extreme climate, it was critical to have an ‘enclosure-first’ approach to ensure energy efficiency and outstanding comfort. A layer of mineral wool encapsulates the mass timber structure to create the thermal boundary and simplify thermal bridge free detailing. A fixed structural module based on CLT sizing is used to formulate the programmatic requirements, sized to allow ease of prefabrication and delivery.
The south façade makes use of building integrated photovoltaic panels (BIPV). Although there is a reduction to the system efficiency with the panels being installed vertically as opposed to at an optimum angle, this configuration eliminates any risk of snow load buildup throughout the winter and serves a double duty as the cladding on this façade.
3. Prefabrication was essential
To negate the challenges posed by the site’s remote location and seasonal construction window, prefabrication was an obvious fit. This was essential to allow for a quick erection of the building during the summer season while decreasing the amount of equipment and materials needed to be delivered to site—reducing the project’s embodied carbon footprint.
A local contractor with passive house experience and prefabrication expertise (BC Passive House) was commissioned to prefabricate building elements off-site. Minimizing site disturbance, the modular prefabricated home is set on a light structure above the uneven terrain. This reinforces its relationship to the site as a ‘visitor’, allowing nature and the site to remain the focus.
4. Off grid self sufficiency
As an off-grid system, the building was required to be self-sufficient. With the added challenge of an extreme climate, the passive house standard was set as a goal with an aim of realising significant energy savings while also creating a comfortable indoor environment. By reducing the operational energy demand, on-site energy generation became feasible to implement.
The building uses solar energy as the main energy source through a 32 kW PV array (103 panels mounted vertically on the south façade) that feeds a 96 kWh battery pack. A storage shed composed of two 20 ft long shipping containers accommodates electrical equipment including a fuel cell, 12 hydrogen cylinders, Tresvolt batteries, invertor and electrical control panels. The 5 kW fuel cell and 120 kW hydrogen cylinders provide back-up power when there is no winter sun or peak use exceeds battery capacity.
In an ideal off-grid system, the batteries would store enough energy to power the development for one day, and then the PV array would generate enough to recharge the batteries each day. Balancing storage, production, and demand without oversizing costly equipment required careful consideration. Satisfying peak electrical demand during the winter using only PV panels and battery storage would require an unreasonably large and costly PV array and battery system, which would be underused in the summer. As a result, the system is sized for average annual demand. In this scenario, the batteries reach their low-level limit more frequently, more efficiently using the capacity of the batteries and significantly reducing the required battery system cost. However, this increases the backup requirements during the winter. The use of a hydrogen fuel cell is currently being trialed to serve as a back-up generator, to minimize carbon emissions in operation.
Complementing the home’s solar generation, the house processes its wastewater and collects and treats its own drinking water. Provisions were also made for the future installation and connection of wind power. In a time of rising energy costs this frugality is equally applicable in an ‘on-grid’ context.
5. Celebrating wood & reducing embodied carbon
Structural materials typically account for approximately half of the material in a building and represent the single largest opportunity to reduce embodied environmental impacts. Materials used in SoLo were carefully designed to maximize their structural capabilities and minimize the project’s environmental impact. Wood was chosen as the primary structural element to reduce embodied emissions. It is expressed and exposed intentionally throughout the project to authentically showcase the types of mass timber products used.
The key structural materials used in the project include:
- 341 m3 of wood: dowel laminated timber (DLT) wall and roof panels, glulam beams and columns, nail-laminated timber (NLT) and cross-laminated timber (CLT) floors.
- 89 m3 of concrete: cast-in-place concrete foundation and footings.
- 3.6 m3 of steel: beams, columns, and decking of the raised platform, and steel rod brace frames.
Post-construction, Perkins&Will and Integral Group undertook a whole-building LCA of the project to understand the embodied carbon associated with structural, architectural, mechanical, electrical, and plumbing (MEP) systems, and on-site renewable energy systems.
When accounting for biogenic carbon using wood within the LCA model, it highlighted that the structural materials were not the largest portion of the project’s global warming potential (GWP). Instead, the architectural and interiors components were the largest contributing category, with insulation being the prime contributor. This is a by-product of taking an envelope first approach using highly insulated assemblies, designing a project with a compact form, and limiting materials such as concrete and steel.
The project team found that the renewable systems (required in an off-grid setting) accounted for the majority of GWP by MEP systems. Batteries are responsible for the largest portion, closely followed by the PV Panels and PV Inverters.
It is a nuanced discussion as there are several overlapping design and performance considerations when it comes to balancing resiliency and occupant comfort. We must consider sizing of mechanical systems, procurement of sustainably harvested wood products and the local energy supplies, i.e., how is energy supplied at a district level? And what will the environmental impact of this infrastructure be as we strive to accommodate a growing population?
Further information on the LCA analysis of the project will be released in the coming months.
6. Certification
In a climate that typically ranges from -30° C in the winter to +35° C in the summer (up to 44° C in the area’s recent heat dome), the difficulty in obtaining the passive house standard is exacerbated. The project location also added a degree of difficulty as the valley has limited solar access due to mountains to the south.
Passive House Planning Package (PHPP), the energy modelling software used for passive house certification, influenced the design both in siting and tendency towards a more compact form in early massing. However, two competing design criteria briefs emerged; firstly, a double height space in the living area that would accommodate a gathering space (for the building’s use as a corporate retreat) with an expanse of glass facing north-east towards the views, and secondly, raising the building out of the ground creating additional exposed soffits.
These conditions placed emphasis on the performance of the building envelope to reach classic passive house certification. It was evident through PHPP modelling that while reaching the 15 kWh/m2a threshold for certification via space heating demand was possible, it would have been to the detriment of other design criteria outlined above.
Third-party certification was still important to validate the approach, and as result, PHI Low Energy Building certification was pursued and achieved instead, blending the goals of design and performance. The project realized a heating demand of 23 kWh/m2a and an airtightness result of 0.6 air changes per hour at 50 Pa. This is the first building certified under the Passive House Institute umbrella within Perkins&Will and is paving its way with lessons learned for current and future projects.
7. Research
SoLo is a prototype. Not just in terms of construction methodologies, but also as a testing ground for improved and optimized design processes.
The project team leveraged an internal innovation incubator micro-research grant to investigate outstanding questions that may not usually have the bandwidth to be investigated in day-to-day project work. In this case, the project team created a research report: “Increasing understanding of the role of thermal bridging in building performance and the design process”, co-authored by Cillian Collins, Alysia Baldwin & Aik Ablimit.
Although successful in achieving PHI Low Energy Building certification (heating demand less than 30 kWh/m2a), this project fell short of achieving the 15 kWh/m2a space heating demand target for full passive house certification. If the lower space heating demand had been met, passive house plus certification could have been achieved due to the level of PV generation applied.
Throughout the project design, the team considered several factors to achieving the lower space heating demand number including:
- How much double height space can we have?
- How much northeast glazing can we have while balancing performance, views, and daylighting? What is the optimum amount of south-facing glazing?
These questions were resolved intuitively throughout the design process with the validation of assumptions through PHPP modelling and juggling the energy balance of the window sizing with heat loss vs solar gain, as well as the balance between window and PV sizing.
However, there is a potential benefit from the power of computational design that would allow designers to review and weigh up the impact of interlocking design parameters on often competing performance metrics such as energy, daylighting, cost, views, etc. This was the topic of another research paper: “Computational design for Passive House projects”, authored by Cheney Chen & Cillian Collins. For more information on this paper, please visit: tinyurl.com/58p8xeat
8. Takeaways
The innovation of SoLo lies in taking technologies that already exist and synergizing them in a way that creates a replicable formula: one that creates high-quality, high-performance buildings that maintain design quality and aesthetics, while focusing on occupant health and wellness.
The future potential of this project lies in bringing all these approaches together and scaling them from a single cabin in the woods to high-rise mixed-use projects in urban centres. By tackling both embodied and operational emissions through the adoption of new construction methods consisting of mass timber, modular/prefabrication, passive house design principles, and clean energy generation, buildings can counteract their negative consequences and act as a source of repair.